A new modeling platform, tested on two recent natural disasters, simulates conditions that dump landslide debris into rain-swollen rivers, often causing more damage than the landslides themselves.
By Peter Lehmann, Jonas von Ruette, and Dani Or
In the spring and summer of 2017, torrential rainfall triggered a series of landslides culminating in two catastrophic disasters: one in Mocoa, an inland city in southwestern Colombia, and another thousands of kilometers away in the coastal city of Freetown, Sierra Leone. The landslides triggered massive debris flows that destroyed neighborhoods and killed several hundreds of people.
Mocoa received 100 millimeters of rainfall within 6 hours, a rainfall that is statistically predicted to occur every 30 years. Freetown received 70 millimeters of rain within 7 hours (based on rainfall data from Climate Hazards Group Infrared Precipitation with Station data, or CHIRPS), a statistical 5-year storm. These two regions are far apart, but they share a tropical climate, and their soils are predominantly clay loam, according to the SoilGrids database.
These intense rainfall events have triggered many landslides in both regions: just upstream from the edge of Freetown and several kilometers upstream from Mocoa. In both cases, however, the main cause of the damage was not the direct impact of the soil mass released downslope, but rather the mobilization of the released material (soil, rocks, and trees) by the river channels downstream.
These tragic events point to a gap in our ability to model at the catchment scale the interlinked processes that cause localized shallow landslides to become debris flows and the pathways such flows take. We present here a new type of model that addresses these links. This model can be applied at any catchment, is freely available, and includes a user interface for ease of use.
Landslides trigger devastating debris flows
Near Mocoa, masses of soil and uprooted trees released by the landslides reached the river channels while the rain-glutted streams were flowing at full capacity. The fast-flowing river carried the soil and trees through the channel network. This debris quickly reached the outskirts of Mocoa, where it destroyed buildings and roads and caused more than 250 fatalities. Articles in local newspapers dating as far back as 2014 had warned of the potential hazards of debris-choked stream channels.
The story was similar in Freetown, where the released soil mass itself did less damage to the town than the subsequent debris flows.
Modeling Interlinked Natural Hazard Processes
We applied the new model STEP-TRAMM that was developed in our Soil and Terrestrial Environmental Physics (STEP) group as part of the research project Triggering of Rapid Mass Movements in Steep Terrain (TRAMM) to simulate landslide triggering and debris flow pathways. The model reconciles the highly localized landslide-triggering processes (on scales from 10 to 1,000 square meters) with a catchment-scale view of hydrologic processes (1 to 100 square kilometers).
The modeling platform offers several distinct advantages over existing landslide models:
-
STEP-TRAMM can be applied anywhere on Earth using any publicly available global databases that offer digital elevation data (e.g., EarthExplorer from the U.S. Geological Survey), forest maps (Global Forest Change), soil textural properties (e.g., SoilGrids), and rainfall data (e.g., Global Precipitation Measurement from NASA). These data sets are easily imported into the model as standard ASCII grid files. A digital elevation model is the minimum requirement to run a simulation; the software includes tools to define vegetation patterns and rainfall data, and it provides hydromechanical properties of different soil types.
-
The model first estimates the distribution of soil depth across the catchment as a function of topography. This is an essential step for constraining soil hydrologic and mechanical processes (forces, loads, and failure events).
-
The landslide-triggering module considers dynamics of local hydrology and threshold mechanics where soil elements may abruptly fail (see below). The resulting landslide pattern is highly localized with relatively few landslides within a large catchment area.
- The released soil mass is routed as debris flows whose path and deposition areas are resolved on the basis of local topography, thus enabling assessment of landscape-scale debris flow hazards.
How the STEP-TRAMM Model works
The model simulates highly localized landslides over a soil mantle represented by hydraulically and mechanically interacting hexagonal soil columns 1–10 meters across [Lehmann and Or, 2012; von Ruette et al., 2013]. This model, like other landslide models, calculates the balance of forces acting on each soil column, where soil strength varies with soil wetting and the presence of roots.
Unlike most other landslide models, STEP-TRAMM goes further, continuously calculating load redistribution among columns whenever the force exceeds a strength threshold. The local hydrology and water content play an important role in determining soil mechanical response—these metrics are calculated from infiltration rates, runoff, and water flow through the soil and along the underlying bedrock.
Within the model, an interconnected group of failed soil columns forms a landslide. The soil mass released in the simulated landslide event is fed into a debris flow runout module that calculates pathways and deposition on the basis of local topography. For simplicity, we opted for an empirical approach [Rickenmann, 1999] for relating runout distance to landslide volume and height difference. This approach provides reasonable estimates of flow paths and runout distances [e.g., Fan et al., 2017].
The main differences between STEP-TRAMM and other landslide hazard models based on geographic information systems are the mechanistic representation of local failure and load redistribution that precede an abrupt landslide, the simulation of catchment-scale soil depth, and the spatially resolved coupling between landslide triggering and debris flow runout pathways.
STEP-TRAMM simulates the Mocoa and Freetown events
We have used the STEP-TRAMM modeling platform and available data to compute landslide triggering and debris flow runout paths for the Mocoa and Freetown 2017 landslide events at a spatial resolution of 10 meters. For the Mocoa event, the simulations produced 80 landslides with a total soil volume of 200,000 cubic meters, and these were transformed to simulated debris flows.
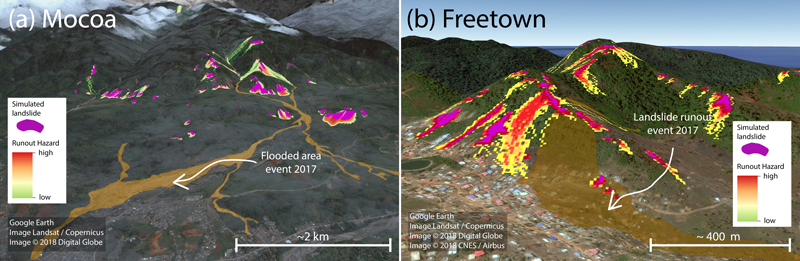
STEP-TRAMM simulations of landslide soil mass releases (purple areas) and debris flow runout (warm colors indicating different calculated probabilities of runout). The brown shapes show the actual debris flow and river areas causing damage, as delineated from satellite images. (a) In Mocoa, many runout paths reached the channels of the river network. Landslide material accumulated in these channels and eventually was mobilized and caused destruction in the town (brown areas). (b) In Freetown, the landslide was transformed into debris flows that destroyed the part of the town at the bottom of the slope.
The simulations show that many debris flows reached the streams at the bottom of the slopes and were subsequently transported to Mocoa. For the Freetown event, the impact of the debris was more direct because the released mass advanced directly toward town.
Satellite images allow us to compare simulated debris flow pathways with the actual path of the damage. The simulations do not reproduce all observed pathways, but they do capture the main features of the real-world event, such as the concentration of landslides in a limited area in the eastern part of the catchment. Global deforestation maps show that several of the patches where landslides originated were deforested sometime between 2002 and 2016, suggesting a potential role of deforestation in promoting onset of landslides in this region.
Outlook and potential applications
Inherent heterogeneity and the complexity of landslide-triggering processes preclude exact prediction of landslide release locations. Our simulations of the two 2017 landslide disasters were entirely based on remote sensing information and educated guesses of initial conditions, without local calibration. Nevertheless, STEP-TRAMM has been able to capture the salient features of landslide release events, including the numbers of individual slides, volumes of material, and approximate locations in the landscape.
The STEP-TRAMM platform offers a means for informed scenario generation, analyses of past events, and creating physically based risk mitigation strategies at the catchment scale. The platform could also be used to generate new science, for example, to systematically study how present and future rainfall characteristics and land use changes (e.g., deforestation) could affect landslide patterns.
Clearly, additional testing across regions and conditions is required, and we hope that the natural hazard community will make use of this publicly available tool to advance process understanding.