Using cave formations to investigate ancient wildfires
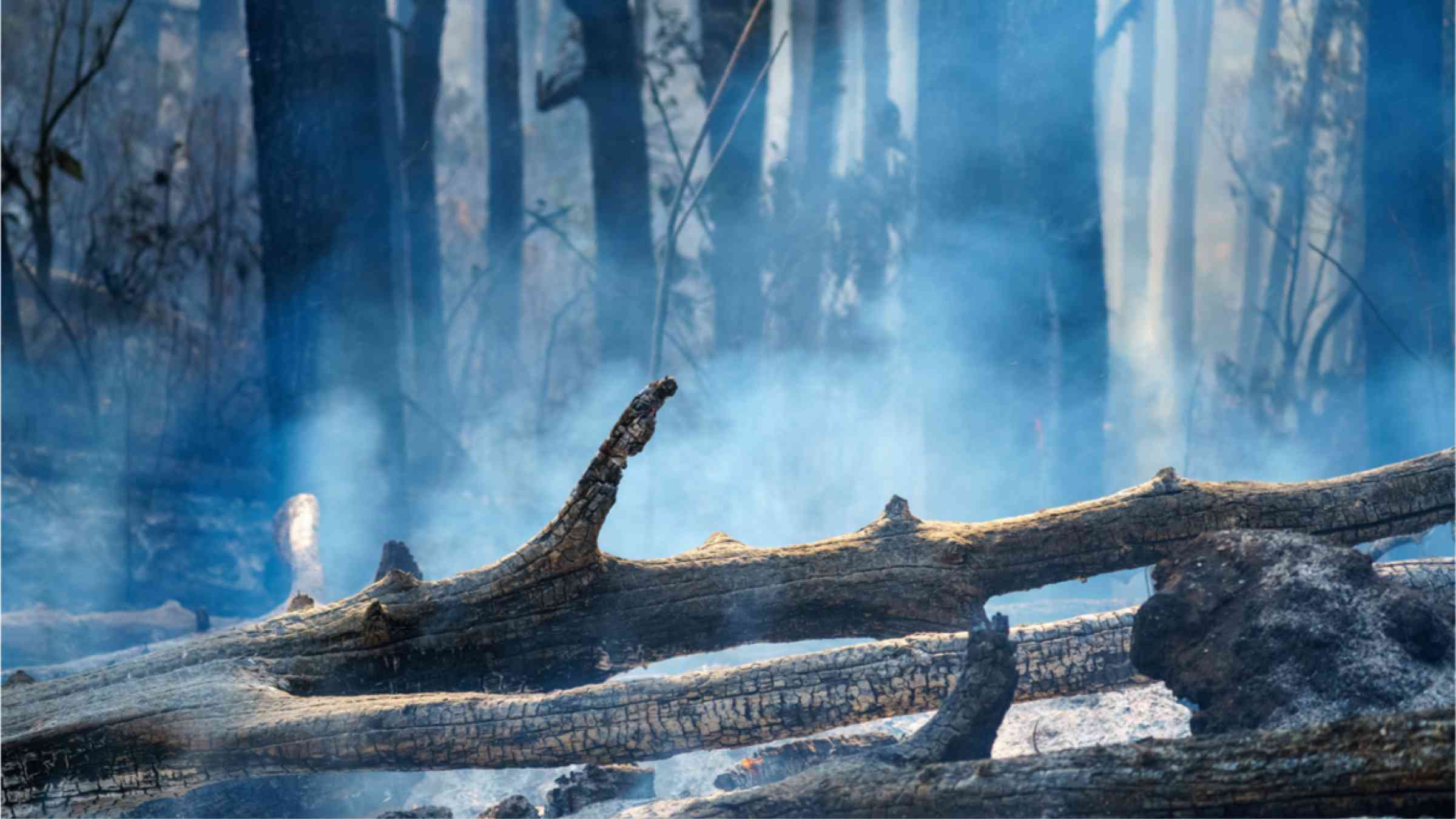
Wildfires affect 40 percent of the Earth’s terrestrial biome, and are likely to become larger, more frequent, and more severe with climate change. Observational records are not long enough to fully characterize changes in fire behavior, but by using environmental archives such as sediment cores, ice cores, tree rings, and speleothems (cave formations including stalagmites), we can look into the past and see how climate and fire patterns have changed over time.
Speleothems are the most recent addition to the paleofire toolkit, and a recent article in Reviews of Geophysics covers the emergence of this new sub-discipline. We asked the authors to give an overview of wildfire regimes, explain how speleothems help us understand them, and outline what is still unknown.
In simple terms, what are the main connections between climate and wildfires, and what effect is ongoing climate change expected to have on the occurrence of wildfires?
Fire regimes, the characteristics and patterns of fires occurring in time and space, are dictated by a combination of climate, human activity, vegetation communities, and feedbacks between them. Climate change has already produced significant increases in fire weather and fire season length (Jones et al., 2022), although this has not necessarily led to increased fire frequency and severity in all regions, as the impacts of increased fire weather can be offset by fire management strategies and vegetation communities.
How can information about fires that occurred in the past help us predict and prepare for catastrophic wildfires in the future?
While modern satellite data products let us observe and monitor fire patterns at very high resolutions, they don’t capture the full range of natural fire behavior.
Our understanding of fire patterns is formed from relatively short observations. While modern satellite data products let us observe and monitor fire patterns at very high resolutions (in both time and space),
Since the interactions between climate, environment, and land management are complex, longer timeseries of past environment, fires, and climate are needed to help us understand these interactions. Improved knowledge of these interactions will allow for better management strategies to mitigate the impact of climate change on future fire regimes.
What are the main sources of information about fires that occurred in the past? What are their main strengths and limitations?
Natural archives such as sediment cores, ice cores, trees, and speleothems incorporate information about the climate and environment as they grow. This information can be used toinvestigate changes in climate and environment over long time periods (hundreds to millions of years) through changes in the chemical and physical properties of the archives. We refer to these chemical and physical variables as ‘proxies’.
Each archive has strengths and limitations. Ice cores can be very long-lasting, but any past fire signal recorded by them tends to be related to mean hemispheric fire activity. Sediment cores are very common, but they can be biased towards large fire events. Fire scars on trees with annual tree rings can give precise timing of fires but rely on the tree surviving the fire. Speleothems can produce long records, be dated accurately, and produce high resolution data, but they are less common than lakes or trees, and hydrological processes can impact the transport of the fire signal.
What kinds of information about past wildfires can be found in caves?
Fire information incorporated in speleothems indirectly comes from the vegetation above the cave and can be thought of as an archive related to ‘you are what you eat.’ Similar to the way our bodies accumulate nutrients from the food we consume, trees above caves bioaccumulate metals as they grow. When these trees are burned, ash containing these metals is deposited on the surface and then leached into the cave system, where it is incorporated into speleothems. At the same time, biomarkers (such as levoglucosan and polycyclic aromatic hydrocarbons) are produced by combustion and transported to the speleothems.
Our team has focused on inorganic proxies because trace and minor elements are relatively straightforward to analyze, and existing analytical tools allow us to measure them at very high resolution. Where the stalagmite growth rate is high enough, these methods allow us to collect data at annual resolution, meaning fire events can be pinpointed to individual years.
How are samples from caves analyzed?
Trace and minor elements are analyzed using laser ablation inductively-coupled-plasma mass spectrometry (LA-ICP-MS) and Synchrotron micro-Xray Fluorescence Microscopy (S-μXRF).
LA-ICP-MS works by using a laser to remove a tiny portion of sample which is ionized in a plasma torch before passing through a mass spectrometer where the proportions of different elements are quantified.
S-μXRF is a non-destructive method which uses X-rays generated by a Synchrotron particle accelerator to bombard the sample. This produces characteristic secondary photons, and by measuring these secondary photons we can determine the sample chemical composition.
Unlike LA-ICP-MS, which typically produces line scans, S-μXRF can produce elemental maps across the sample. In speleothem fire research this is useful, as the metals are not always deposited evenly across the speleothem growth layer, and might be missed by a LA-ICP-MS line scan.
What have some of the samples that you collected in Australian caves revealed?
Our group’s research has shown that fire events are linked with peaks in various metals in speleothems. The figure below is an example of this. A known fire which passed over the cave in 1961 coincides with peaks in zinc and iron.
At a different location, Yonderup Cave in southwest Western Australia, McDonough et al. (2022) found that the timing of peaks in various combinations of phosphorus and metals including zinc, aluminum, lead, and copper within a stalagmite coincided with all known recent fires. They used this information to reconstruct about 250 years of fire history for the cave and showed that an intense wildfire in the late 1800s (the early colonial period) followed an extended period of drought. This drought may have exacerbated changes to land management after Indigenous cultural practices were stopped, resulting in the intense fire.
What are some of the unresolved questions where additional research, data, or modeling are needed?
A key current research question is whether past fire severity (rather than just frequency) can be inferred from speleothem geochemistry. This would enable reconstructions of past climate, fire frequency, and fire severity from just one proxy archive, which would be a significant step forward in understanding climate-fire interactions.
Our research has also shown that fire can impact the speleothem δ18O signal due to partial or complete evaporation of water in the unsaturated zone above the cave. δ18O is the most commonly applied speleothem climate proxy, and has been extensively used to reconstruct past climate. A comparison of the Speleothem Isotopes Synthesis and Analysis (SISAL; Comas-Bru et al., 2020) database with MODIS Burned Area data (Giglio et al., 2015) showed that in recent decades about 50% of SISAL sites were burned, meaning that many speleothem paleoclimate records may have been affected by fire. More research is needed to determine the extent of that effect.